The word atom comes from the Latin for indivisible. But don’t let the name fool you.
A simulation by US theoretical physicists has provided the first fully microscopic characterization of the moment an atom splits in two, revealing new insights into an energetic event that came to define a new era in science and technology.
In 1938, we found out how wrong that little word is when physicists Otto Hahn, Lise Meitner, and Fritz Strassmann showed how uranium nuclei split in two when they were shot with neutrons.
Decades later, despite its use in war, power, medicine and scientific investigation, nuclear fission is slow to give up its secrets.
Beyond simple patterns of protons and neutrons packed together like bubblegum in a diffuser, the nucleus of a massive atom is a wild storm of quantum activity.
Understanding how individual nucleons behave and interact with each other is challenging enough for atoms that stand quietly by their lonesome, let alone for those that undergo significant transformations.
To make it easier to follow, theoretical physicists from Los Alamos National Laboratory and the University of Washington (UW) break down the fission process into four steps.
In the first 10-14 seconds (give or take), the introduction of a slow-moving neutron forces the nucleus to swell and rearrange into what is described as a saddle point, making the atom look a bit like a tiny peanut shell.
This is quickly followed by a much faster shift, referred to as the incisional saddle, where the fragments of the fission process settle down. This takes about 5×10-21 seconds.
The third step is again even faster, transforming to a relative blink of 10-22 seconds. In what is called cutting, or neck snapping, the core is officially separated.
In the last step, who gets a lazy 10-18 seconds to unfold, the fission fragments are pulled back into shape and accelerated, releasing neutrons and gamma rays and potentially generating other decay processes after a short delay.
More than one theory describes the exact migration of sub-atomic particles from peanut to pop, although in many cases experimental results either contradict basic assumptions about physics, or contradict ‘microscopic’ modeling of the interactions between individual protons and neutrons.
Based on a framework developed by lead author UW physicist Aurel Bulgac, the quantum many-body simulation is the most accurate description yet of what to expect at the precise moment of shear, when the bridge connecting the two halves of a large atomic nucleus snaps . in and divides.
Calculations on uranium-238, plutonium-240, and californium-252 under various initial conditions made extensive use of the US Department of Energy’s Oak Ridge National Laboratory supercomputer.
“This is probably the most accurate and careful theoretical description of neck snapping, without any assumptions or simplifications,” says Bulgac.
“We have a very specific prediction, which until now did not exist. Previous theories were always based on, ‘Let’s assume this is happening, and if it is happening, then this will probably be seen.’ We didn’t do that. We just put in the equations of motion known for many decades in high-precision nuclear physics, plus quantum mechanics, nothing else.
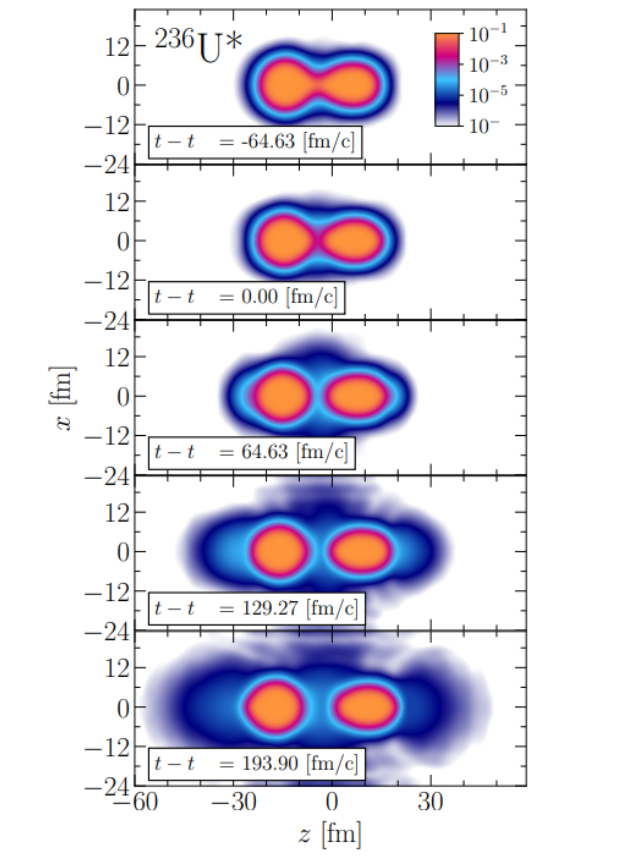
The simulation revealed some surprises in the separation process. Where some models had predicted a generous dusting of quantum randomness in the neck snapping process, the team’s model identified a clear ‘wrinkle’ in the density of subatomic particles that preceded the appearance of the cut point.
There was also a marked difference in the splitting time between the two types of nucleons, with the proton neck completing its break before the neutron neck.
Crucially, the simulation confirmed controversial proposals of a release of highly energetic neutrons during the shear phase, with the model going so far as to predict their energies, angular distributions and even escape directions.
“Most experiments look for them in the direction of motion of the fission fragments, and they couldn’t detect the shear neutrons there because most of them were thermal neutrons emitted by the hot fragments,” says Bulgac.
With the predictions in hand, the next step is to see if experimentation supports these recent discoveries of how the ‘undivided’ atom splits into two.
This research was published in Physical review papers.